Decoding adult stem cells: exploring the hallmarks of stemness
Published by HUB Organoids on Feb 19, 2024
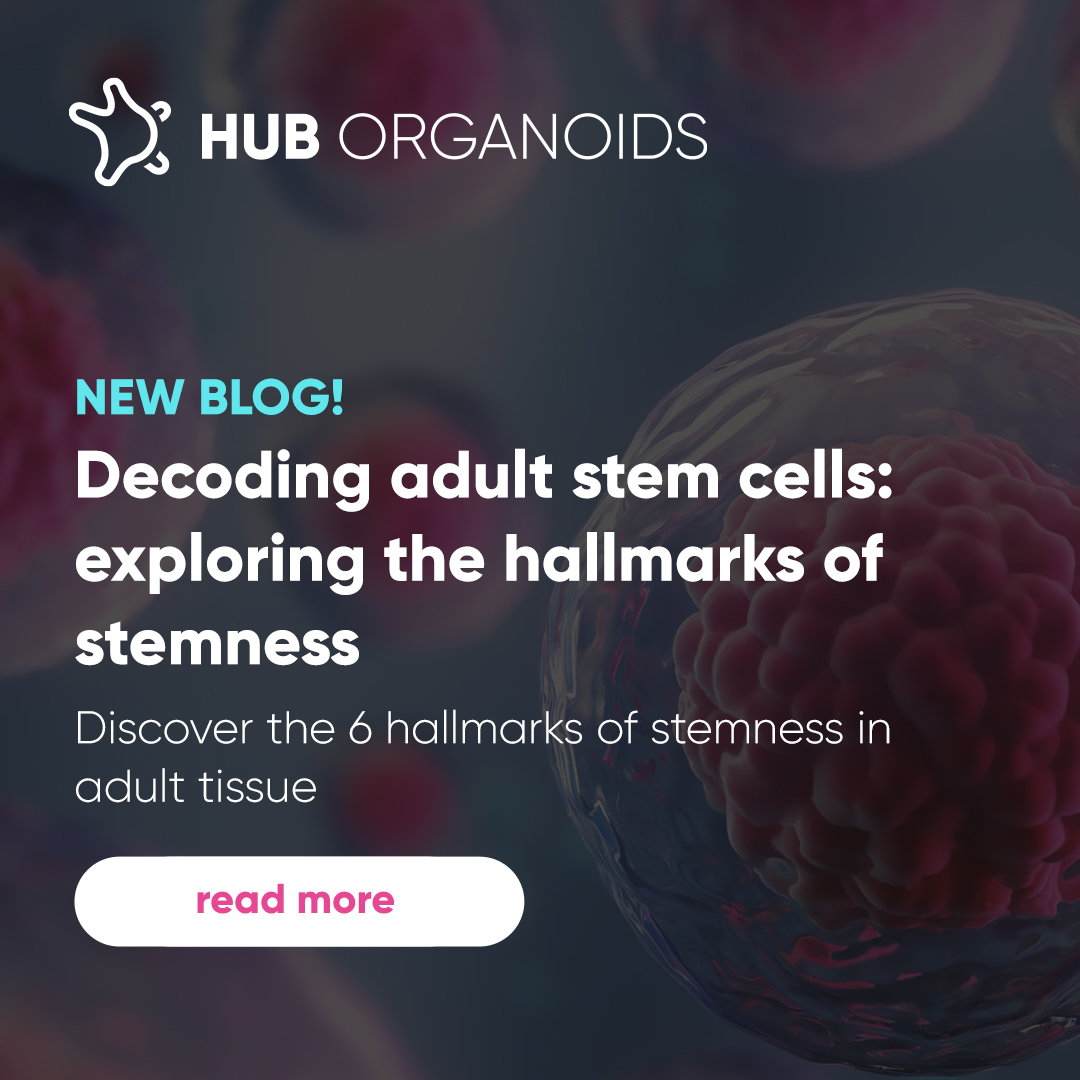
Discover the 6 hallmarks of stemness in adult tissue
Mammalian organ systems face a constant barrage of internal and external stressors, leading to cellular attrition and functional decline. Maintaining tissue homeostasis in this dynamic environment requires a coordinated response from diverse populations of adult stem cells (ASCs). Recent years have witnessed a paradigm shift in our understanding of ASCs, revealing a remarkable heterogeneity in their repair strategies across different tissues.
This blog delves into the intricate world of tissue-specific ASC biology, dissecting 6 key hallmarks that define their regenerative potential. We explore the unique niches they occupy, their distinctive modes of self-renewal and differentiation, and the specialized mechanisms they employ to restore tissue integrity and function. By unveiling the diverse toolkit of ASCs, we aim to shed light on the remarkable adaptive capacity of mammalian organ systems.
Figure 1: Infographic depicting the six key hallmarks of stemness in adult mammalian tissue
Hallmark 1: Self-renewal and population longevity
Arguably the most important trait of a stem cell population is to persist over the lifetime of the animal. Two things are crucial for this: protection against DNA damage, and a balance between retaining stem cell population and differentiation into mature daughter cells. Historically, the asymmetric division model, wherein a stem cell division yields both a stem cell and a daughter cell through intrinsic cellular factors, was thought to be the exclusive mechanism for self-renewal in the ASC population. While instances such as Drosophila neuroblasts support this model, numerous solid tissue ASCs sustain their lineage through an alternative mechanism known as symmetric division. The symmetric model proposes that a stem cell generates equipotent daughter cells, and based on their position in the stem cell niche and their interaction with niche microenvironmental cues, they remain as stem cells or commit to differentiation. Rather than individual stem cells persisting long-term, the niche
size imposes space constraints, thus strictly controlling the number of potential stem cells. This form of competition is termed neutral drift, which leads to clonality.
Imagine a game of musical chairs where players represent stem cell clones in a niche. As the game progresses, chairs are removed randomly (neutral drift), causing some players (stem cell clones) to leave the game while others remain seated. Over time, certain players (stem cell clones) occupy more chairs and become dominant, while others fade away. Therefore, the game's longevity (or outcome) isn't determined by any individual player (stem cell), but by the dynamics of the entire group (stem cell population).
In tissues like intestinal epithelium, stomach epithelium, and skin appendages which have a higher demand for cell repair and renewal, the neutral drift and symmetric model of division ensures a constant shuffle of fresh cells. Unlike Drosophila neuroblasts, it is about the population's longevity, rather than that of individual stem cells.
Hallmark 2: Multipotency
The multipotency of stem cells, which is the ability to generate diverse cell types, exists as a dynamic spectrum rather than fixed categories. It includes both the steady dedication to lineage maintenance during normal conditions and the latent adaptability revealed during tissue repair or regeneration. This plasticity is governed by transcriptional and epigenetic programs responding to niche cues.
Under usual conditions, many tissues rely on a single type of stem cell to generate various cell types, such as in the crypt-base-columnar cells in the intestine or satellite cells in skeletal muscles. However, the murine hairy skin stands out. It's like a continuous sheet with different sections, each hosting its own set of stem cells. Interestingly, these stem cells aren't confined to their specific section; they exhibit a remarkable ability to move between sections and fulfill different roles when the skin is damaged. This adaptability becomes evident when individual hair follicles are transplanted and contribute to repairing other areas of the skin.
Functionally specialized multipotent cell subpopulations within tissues represent an evolutionary marvel of survival. They synergistically contribute to regeneration through both compartmentalized and cross-compartmental lineage commitment, showcasing nature's ingenious strategy for maintaining tissue integrity and resilience.
Hallmark 3: Transplantation
Before the development of more advanced tools for studying development, scientists relied on a basic test to prove the existence of stem cells. In the 1960s, researchers McCulloch and Till created a test called the "spleen colony-forming unit." They checked if bone marrow cells transplanted into the spleens of damaged mice could save them. They found that the transplanted spleens grew clusters of cells from a single transplanted stem cell, estimating that fewer than 1 in 1,000 bone marrow cells could do this. Since then, similar tests have been developed for almost all types of adult stem cells. However, not all stem cell types show this transplant success, and the test has limits in showing how well stem cells can multiply in a new environment.
However, it is worth noting that the ability to transplant cells has been crucial in identifying cancer stem cells. This concept, pioneered by John Dick in the 1990s for blood cancers, suggested that tumors have a structure similar to the hierarchy of stem cells found in their original tissue. A key way to confirm this theory was by sorting cells from a primary tumor and then seeing if they could grow cancer again when transplanted into mice with weakened immune systems. This approach has been the basis for many alternative models in cancer and immunology research.
Hallmark 4: Plasticity
Stem cell identity extends beyond a fixed lineage. This can be further explored through Waddington's landscape, which illustrates how cells transition from an undifferentiated state to various distinct cell fates during development. During homeostasis, cells progress through commitment and differentiation as they move away from the stem cell zone and no longer have access to stem cell niche factors. However, when damage occurs and stem cells are lost, most crypt cells can easily revert their commitment when exposed to stem cell signals.
In rapidly renewing tissues like the gut, damage activates "de-differentiation" even in committed progenitors, allowing them to revert to multipotency and contribute to regeneration. The liver, lacking dedicated stem cells, exhibits diverse plasticity. Hepatocytes ramp up proliferation, while chronic injury induces bipotent "oval cells" from cholangiocytes. This spectrum highlights how tissues deploy distinct plasticity strategies, dependent on turnover rate and niche cues, showcasing the remarkable resilience of stem cells and mature populations alike.
Figure 2: Illustration depicting the Waddington's landscape
Hallmark 5: Niche dependence
The mysterious stem cell niche, a concept first proposed by Ray Schofield in 1978, plays a significant role in determining the fate of stem cells, much like the highest point on Waddington's landscape guiding cellular outcomes. Understanding its complexities has been a long-standing challenge for stem cell researchers.
Traditionally, techniques like immunohistochemistry and in situ hybridization provided limited insight into the intricate signals within the niche. However, recent advances have revolutionized niche research. Single-cell RNA sequencing now offers detailed maps of potential signaling interactions, helping to reconstruct niches in the lab. Spatial transcriptomics provides high-resolution views of signaling patterns, while advanced cell isolation methods help identify specific niche components.
The relationship between the niche and its resident stem cells goes beyond simple signaling; it's more like a dynamic dance where both partners influence each other. For example, maturing keratinocytes affect the underlying pool of basal stem cells by becoming more contractile. In hair follicles, there are two types of stem cells: "primed" ones ready to divide and "quiescent" ones resting. They work together in the hair growth cycle. When primed stem cells divide, their immediate offspring produce a protein called sonic hedgehog, which is important for activating quiescent stem cells. These resting stem cells can then create more primed stem cells, ensuring the continuous renewal and growth of the hair follicle.
Hallmark 6: Maintenance of Genome integrity
The stem cell niche, beyond influencing lineage choice, offers crucial protection against harmful mutations. While quiescence isn't the sole answer, stem cells employ diverse strategies to maintain genomic integrity.
Hematopoietic stem cells (HSCs) heavily rely on non-homologous end joining (NHEJ) to patch up damage. Hair follicle stem cells employ a robust anti-apoptotic shield, ensuring survival after DNA damage. In the gut, Cryptic base columnar (CBC) stem cells express many homology-dependent repair (HDR) genes, further bolstering error-free repair.
Beyond repair mechanisms, metabolic adaptations provide additional layers of protection. HSCs, for instance, operate on a low-glycolysis engine, minimizing the production of reactive oxygen species (ROS) known to damage DNA.
The number of stem cells in their environment also matters. In places like intestinal crypts where there are only a few stem cells, damaged cells can easily be removed without causing harm. But in places like the bone marrow where there are lots of stem cells, mutated cells can multiply more easily. Additionally, stress-induced differentiation or de-differentiation might serve as safeguards, replenishing healthy stem cell populations to outcompete mutated counterparts. However, oncogenic mutations promote rapid clonal expansions of mutant stem cells and can be detrimental. Understanding how the stem cell environment, the immune system, and the way cells grow interact is still a big challenge, especially when it comes to cancer.
Summary
The exploration of tissue-specific adult stem cell biology has uncovered six key hallmarks that delineate their regenerative potential. This nuanced understanding reveals a sophisticated interplay between stem cells and their microenvironment, emphasizing the dynamic nature of cellular repair strategies across diverse tissues. From the intricacies of self-renewal and population longevity to the multifaceted nature of niche-stem cell relationships, the field has witnessed a paradigm shift. Multipotency, transplantation, plasticity, niche dependence, and genome integrity maintenance further underscore the adaptability and resilience of stem cells. These insights hold significant implications for developing novel therapeutic strategies to address tissue aging, injury, and disease. By deciphering the remarkable toolkit of adult stem cells, researchers are poised to unlock new avenues in regenerative medicine, offering promising prospects for the future of tissue repair and maintenance in mammalian organ systems.